For many years it was difficult for people to understand the complex nature of heat transfer through wet thermal insulation. Figure 1a shows one of such measurements. Figure 1a shows that the relation of thermal conductivity on moisture content of the material depends on period of the measurement. Undoubtedly, presence of thermal gradient changed the distribution of water in the material and some engineers, (Figure 1b) decided that doing a rapid test.They assumed that as the rapid test does not include movement of moisture it may give us a real effect of water on thermal conductivity.
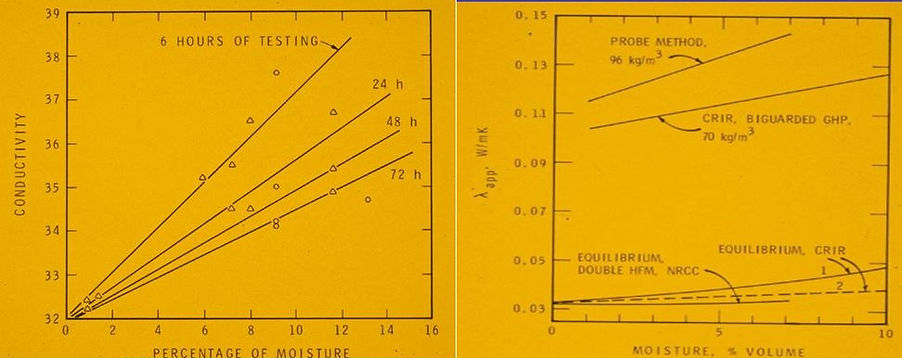
Figure 1a Thermal conductivity of fiberboard measured in 1932 as a function of moisture content and the duration of the test.Figure 1b: Comparison of rapid test methods with equilibrium testing at the same moisture content in different laboratories
Figure 1b shows that using the rapid measurements does not reduce the effect of moisture. A contrary effect of increased thermal conductivity has been obtained with a probe method and somewhat similar is an effect obtained the constant thermal gradient method (Guarded Hot Plate) method. Thus, the issue warrants a careful examination.
Figures 2a, 2b and 2cshow the preparation of a MFI (mineral fiber insulation) specimen and Figure 3a and 3b show the moisture movement and the continually measured thermal conductivity coefficient by CRIR (Center of Industrial Research in Rantigny) of St.Gobain company.
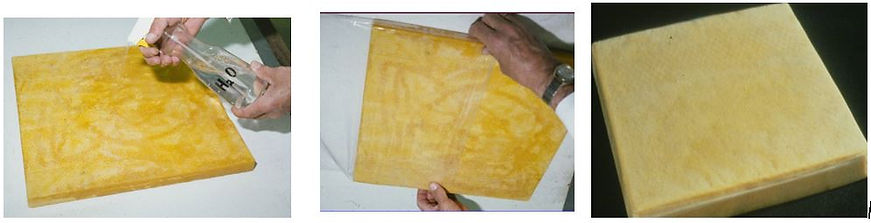
Figures 2a, 2b,2c: Preparation of MFI specimens for testing.

Figure 3a: Schematics of moisture redistribution in a specimen tested for the thermal conductivity coefficient. Figure 3b: thermal conductivity coefficient measured at CRIR on two wet MFI specimens.

Figure 4a and 4b: A comparison of MFI (left) and CFI specimens (right).Figure 4a shows heat flux versus test duration and Figure 4b moisture distribution profiles at the dynamic equilibrium. Test performed at NRC Canada laboratory.
Figures 4a an 4b shows that the pattern of phenomena is similar yet differences in the effect of water on heat transfer through the material. In mineral fibers, water is concentrated in a narrow layer near the cold surface and the effect on the heat flow is small. Conversely, the water transfer in the cellulose fiber insulation is slower and water is spread over wider range of the material making effect on thermal conductivity somewhat larger. The difference is caused by the cellulose fibers being more hygroscopic than mineral fiber.
The effect of water flow on heat flow depends on the rate of water vapor flow in the material and the value of thermal gradient because the phenomenon includes two independent components. Water vapor(WV), driven by the thermal gradient, causes condensation of WV and then the condensed water moves towards the dryer material. These two processes last several hours and at the end a dynamic moisture equilibrium is reached. We are talking about dynamic equilibrium (or a quasi-steady water profile) because two different transports occur at each place at each moment of time. The thermally driven water vapor transport goes towards the cold side and liquid water equalization goes in the opposite direction. Therefore, one of the popular names of this phenomenon is a thermal syphon.
The intensity of condensation depends on local temperature in point of condensation so the S-shaped curve for each value of thermal gradient will look different if the mean temperature is different, e.g. the same test is performed with different thickness of the test specimen. The rate of water vapor transports(WVT) are measured differently in the EU and NA. In EU one uses so called miu-value as a function of moisture content, i.e. ratio of the actual WVT to a diffusion through air layer. In North America one uses actual values of the transport coefficient.
Effectively, one may consider water as phase changing material (PCM) and consider two independent effects: (a) presence of immovable water or PCM as any disperse (immovable) phase in the matrix of tested material, and (b) movement of water a rate approximated by the WVT coefficient.

Figure 5a and 5b: Comparison of a Spray Polyurethane Foam (SPF) (left) and Extruded Polystyrene(XPS) specimens (right). Figure 5a shows heat flux versus test duration and Figure 5b moisture distribution at the dynamic equilibrium. Test performed at NRC Canada laboratory.
As both foam products are not uniform, the profiles of the dynamic moisture equilibrium within the specimen, shown in Figures 5b,are different. The extruded polystyrene has a uniform material core but different density on the surface and as the rate of water vapor flow is much slower at the surface layer one can see water accumulation there. We do not see water in the material core because the foam plastic has a negative wetting angle and repels water accumulation.
On the other hand, a moisture profile in the SPF shows a beginning of the expected S-shape curve but reaching a peak in the middle of the layer and a low slope towards cold side. One must remember that the SPF specimen has mostly closed cells and that the specimen is sealed on both sides. Reaching maximum at the cold surface the resistance to the thermally driven moisture flow is growing and the water content gradient is getting steeper while it moves further towards the low temperature. At a certain moment both vapor and liquid phase flows unite to carry more water vapor towards the cold side. As SPF contains mostly closed cells with a small volume of open cells, the small water content gradient is sufficient to distribute the free capillary water.
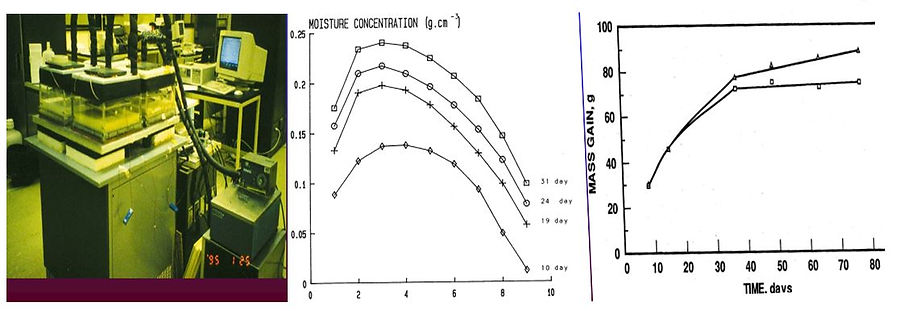
Figure 6a: Test set-up where specimen is set in the air between hot water and water absorber on the cold side. Figure 6b: Moisture profile in the Spray Polyurethane Foam (SPF) with both surfaces opened and thermally driven water vapor entry. Figure 6c: Mass of water entering form the hot water side versus time. Test performed at NRC Canada laboratory.
Figure 6b shows measurements of moisture content distribution during diffusion of water with both surfaces open and indeed we have obtained an asymmetric bell curve, because the surface layers of the foam have larger fraction of open cells than the middle core. Effectively, understanding of moisture transport in foams explains the resistance to freeze-thaw damage in winter.
Figure 6c shows the inflow of water to SPF and polyisocyanurate foams. The rate of water entry to the SPF and polyisocyanurate are slowing with time and even stops within a few-week period, indicating that an increase of moisture during the winter exposure will not result in a material water saturation.
In conclusion, one must understand details shown on Figures 3 and 4 because that explain performance of phase change materials (except there the range of changes do not relate to space but to the efficiency of melting and solidifying of the PCM. In turn, Figures 5 and 6 explain freeze-thaw durability of closed cell foams.
Now, we need to broaden the scope of our examination (Figure 7),and ask if changing the boundary conditions would also modify the response of different foam types to water entry. Measured results are shown on the chart in the actual scale but without numerical values because the typical tests used by engineers in Europe or USA do not show the real moisture content inside the material. These tests show an apparent water absorption with water attached to the surfaces i.e., the results depend on the test method used. Nevertheless, the charts represent the measured apparent characteristics. For the SPF the worst case was the constant thermal gradient and thanks to the slow process the moisture pick during the daily oscillation is much slower and the closed cell nature makes it even smaller for isothermal conditions.

Figure 7: Effect of changing boundary conditions: (a) constant thermal gradient, (as Figures 4- 6, (b) freeze-thaw, oscillating gradient, (c) isothermal conditions (constant temperature). Materials: EPS low density (PS 1), medium density(PS2) and SPF (PU).
For expanded polystyrene the results confirm what we have already discussed in the column 1, namely the best performing in exposure to wetness is the low-density material. The denser material picks more water under thermal gradient than under isothermal water intake. One does not need to test water absorption of open cell polyurethane foams, with a very fine open pore structure they behave exactly as denser polystyrene towards water. If water is not a concern, these foams can function as air flow retarder. Note that as long as many of academic people do not want to use open cell foams for air barrier application, one must require results of dimensional stability test when open cell foam is exposed to water condensation.